How would you navigate in space?
26-07-2019
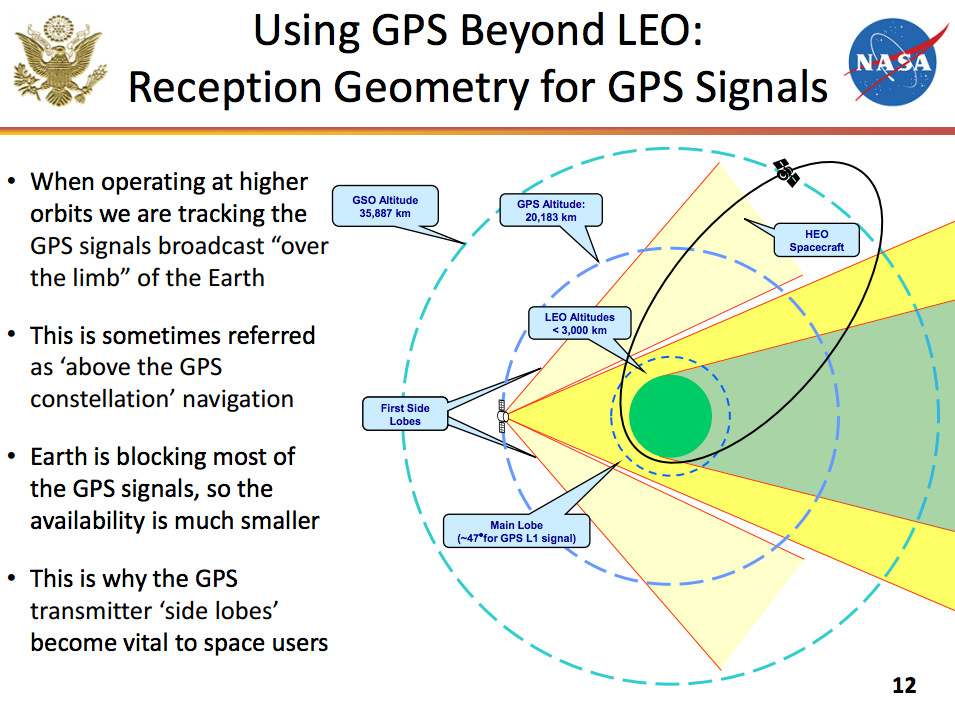
We often have interesting technical discussions in the office - the team all have degrees in technical subjects, across a broad range of subjects, so conversations spring from all kinds of sources. In this case, we had an undergraduate physicist in the office, called Abi, who is specialisng in Astrophysics; Jeff and Tom are both sailors and expert in navigation, so the question arose : How do you navigate in space?
It's an interesting question. On Earth it's largely solved by using a GPS system. Some people keep their hand in by using bearings and even sextants. So, unsurpisingly, this is how they do it in Space. Even a sextant can be useful.
Sextants measure the angular distance between two different objects — usually distant stars. This still works in Space, between the Earth and the Moon. In 1969, a sextant was used for navigation to the moon on the Apollo missions. The sextant combined two separate optical devices that worked in conjunction as a functioning sextant: a 1x wide-field telescope (which was used to identify a target constellation or a single star) and a 28x telescope (which was used to make the actual angular measurement).
The sextant could be used to compute the spacecraft’s altitude and position; whereas in transit between Earth and the Moon, it could be used to compute the spacecraft’s attitude (orientation), position, and velocity. A proper attitude during the flight to and from the Moon was critical for accurate course corrections.
So how would one orientate in the vastness of space if say, all communications were to suddenly drop? A Galactic Coordinate System (GCS) was first described by William Herschel in 1785, a famous British astronomer. It defines the centre of measurement as the Sun, onto which the galactic latitude and longitude is imposed. The galactic longitude of an object is the angular distance around the Galactic equator from the Galactic centre at l = 0⁰ (towards the constellation Sagittarius) to the object.
Further reading on the subject can be here.
Fortunately in the modern era, space craft can for a period use a positioning system (be it GPS, GLONASS, Galileo et al.) to accurately track their ascent into low-earth orbit (LEO). Even whilst in orbit, craft and the ISS use and rely on tracking instrumentation to correct their orbits, dock with external vehicles and payloads and to monitor their position relative to Earth. This is because they are inside of the geostationary GPS satellites.
Since there’s no GPS beyond LEO, navigators process the radiometric tracking data received from the Deep Space Network (DSN) to determine the spacecraft’s position and velocity via a process called ranging. They send a ping to the spacecraft, which responds as soon as it can. They also use optical data, where the spacecraft takes a picture of the star background to help refine the spacecraft’s trajectory.
The DSN consists of a network of extremely sensitive deep space communications antennas at three locations: Goldstone, California; Madrid, Spain; and Canberra, Australia. The strategic placement approximately 120 degrees apart on Earth’s surface allows constant observation of spacecraft as the Earth rotates.
The time it takes for the probe to respond determines it's exact range, much like pinging a server or another computer on a network. If you have its range for a few time samples, and you know the gravitational constants of everything, you can determine what trajectory the spacecraft is on. This is a tried and tested method, used for example with with the Voyager probes and New Horizon.
One of the key components of accurate navigation uses models of stellar dynamics, that is mapping the solar system, the stars and all bodies within a given range as best as is allowed, which in turn creates models of the forces acting on a probe and orbital dynamics, estimating its location.
For example, Cassini's location was typically determined to a kilometer or less relative to Saturn. Using a probe's known position and velocity, its future positions could be worked out. Navigators compare these positions to the predicted location of the target body to determine when a probe will reach its target.
Then, all that's left to do is to collect the flyby data, take a deep breath, and go on to the next encounter.
But that's a large planetary body that can be seen with the naked eye, during
good conditions on Earth.
How about aiming for comet, 4.3 by 4.1 km at
it's largest area, 187 million km from the sun, on a 10 year mission spanning
~750 million km? Welcome Rosetta, a European Space Agency built probe that
attempted a landing on the comet. It deployed it's lander Philae, which was to
shoot two harpoons into the soft surface of the comet.
Whilst this the thrusters and harpoon failed to activate, the probe bounced and settled in a shadowy precipice. For a few days it continued to transmit data and readings which proved to be a unique insight into these icy time capsules scattered throughout or Solar System.
This incredible feat of engineering, coupled with unparalleled mathematical precision and modelling would not have been possible without DSN our ability to precisely calculate relative positions within a couple of km in deep space.